Sun-activated Materials to Purify Water: Photocatalysts
- ENV Tech Blog
- Jul 22, 2023
- 5 min read
Updated: Dec 26, 2023
Scientists look to the sun for sustainable water purification
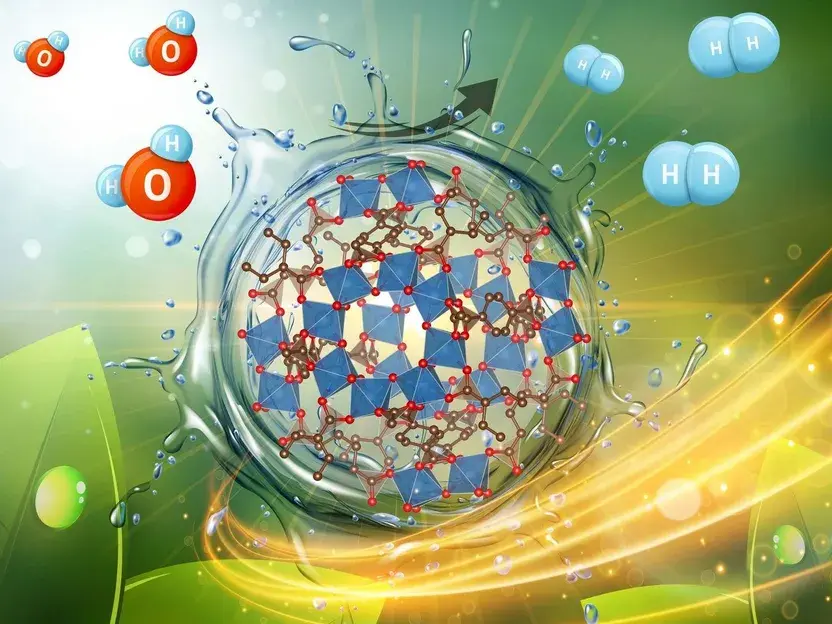
Scientists discovered a material that can sustainably and efficiently purify water under normal sunlight conditions.
More than 2.5 billion people in the world, especially those in underdeveloped / developing countries, lack access to clean water. In 75% of those cases, they drink water that is contaminated with fecal matter, leading to the transmission of numerous diseases from cholera to typhoid. In 2022, water-related issues killed 3.4 million people. This is often due to the lack of regulation of water quality in developing countries since water treatment options often require high capital and maintenance costs.
To much of society, these numbers are just another statistic. But for the family members of those suffering and deceased, the feeling of helplessness is the hardest thing to bear. There are quite a few charity organizations such as Water4 out there working to address the drinking water problem. However, that isn’t the subject of this article.
But here's the good part: imagine if I told you there was an inexpensive material that, under normal sunlight conditions, is capable of destroying contaminants in water. Yes, I wrote that correctly. Not only that, this material is reusable, isn’t toxic, and doesn’t require any special equipment or power consumption to operate.
This type of material is known as… photocatalysts.
Photocatalysts:
With rapid progress being made in the field of photocatalysts through research endeavors, it is imperative to learn what's behind it:
The term “photocatalyst” might sound complicated. But let’s break that down. Essentially, the word “photocatalyst” has 2 components. First, “photo” is the Latin root meaning “light.” The second, “catalyst” is a substance that can speed up a chemical reaction without changing itself. The good thing about science is their terms are quite literal!
So what materials are photocatalysts made of? Here comes the crazy part: they aren’t some mysterious material made in a million dollar machine. The most common photocatalysts are just specific semiconductors and metal oxides.
Semiconductor: is regularly an insulator, but can conduct electricity under certain circumstances, ie. higher temperatures or energy input.
Metal oxides: are compounds that have a metal that is chemically combined with oxygen. For example, rust, iron oxide, is a metal oxide (Fe2O3). Many metal oxides are semiconductors.
Some of the most common types of photocatalysts include: Titanium oxide (TiO2), Zinc oxide (ZnO), Bismuth-based Oxides (BiOCl), and graphitic carbon nitride (C3N4). However, many new materials are being researched and tested for their photocatalytic properties
How do they work?
Now that you know photocatalysts are metal oxides and semiconductors, it raises the question: why these materials?
See, both semiconductors and most metal oxides, when used as photocatalysts, have the ability to create reactive compounds that will destroy contaminants surrounding it.
Prepare:
The first step of this process is putting the photocatalyst in the contaminated water. This puts the photocatlyst in contact with the contaminants so it can destroy them. As the particle size decreases, the effectiveness increases due to increased surface area. Due to this, many times, the photocatalysts are in nanoparticle form.
Excitation:
In a photocatalyst, there are 2 “bands” or areas where electrons can reside. The regular band is called the “valence band.” The higher energy state band is called the "conduction band." When energy from the sun, a photon, with enough energy collides with an electron from a photocatalyst, the electron absorbs this energy and “excites” itself to the higher energy state or the “conduction band.” Here, the electron can move freely and react with compounds nearby.
Generation:
Now here comes the interesting part:
The separated electron (e-) and its corresponding hole (h+) can react to form reactive species. Common pathways:
e- + O2 → O2- (superoxide)
h+ + OH- → • OH (hydroxyl radical)
Degradation:
The reactive species formed above take it from here. Over the period of a few hours, they are able to break down the organic pollutants such as (ie. pharmaceuticals, dyes, and petroleum into harmless water and carbon dioxide. Additionally, they can remediate heavy metals in the water.
Reuse:
After degrading the contaminants of the water, they can be processed for reuse. Usually, this involves laying them out in the sun to dry.
Applications:
Now it might seem like photocatalysis is the best solution for drinking water problems and should be applied immediately. However, like many new and advanced technology, there are some limitations and problems that must be understood and researched. In this case, a main problem is that the conversion efficiency of an electron-hole pair into reactive species is quite low since the electron-hole pair attracts each other and will "recombine" quite fast. Research such as single-atom catalysis and co-catalyst loading hopes to address this problem by moving these precious electrons as far away as possible.
Therefore, photocatalysts have experienced limited application in the industry and the real world for water treatment. In 2015, Panasonic announced its earliest version of their photocatalytic water purification device. It consisted of a titanium oxide photocatalyst inside and UV light produced by a UV lamps from within. Since then, however, there were no further updates, meaning the technology might not have been able to compete with methods already well-researched. In order to prepare photocatalysts for the real world, further research in materials design, degradation efficiency, and cost effectiveness is being conducted to address their limitations.
Here is a comparison of photocatalysts to other water remediation methods:
| Description | Pros | Cons |
Ultrafiltration | A membrane with tiny pores (20 nanometers diameter) that passes water through to purify it. | Removes:
| High synthesis and maintenance costs, pores get clogged over time, and it requires energy. |
Reverse Osmosis | Contaminated water is pushed through a semipermeable membrane (0.1 nanometer pores) to filter contaminants, including salt ions. | Removes:
| Extremely high maintenance (skill and time) required, 1:4 ratio of clean to wastewater, and requires high operating pressures and high energy consumption. |
Chlorination | A compound containing Chlorine, Cl, such as Chloramine is placed in the contaminated water. This can be in pellet or solution form. | Removes:
Does not require external equipment or energy. | Requires commercial chemicals. Only removes viruses and bacteria. |
Biosand / Activated Carbon | Contaminated water is passed through a filter made from biosand and/or activated carbon. They have the ability to filter out contaminants while also “adsorbing” many of the contaminants. | Removes:
Low cost, no energy. | Does not remove bacteria and viruses. Must be replaced often. Cannot remove salt from saltwater. |
Photocatalysts | Produces reactive species like hydroxl radicals and superoxides under light irradiation. | Removes:
No energy, re-usable, no specialized equipment. | Cannot remove salt from salt water. Works worse in bad weather conditions. Limited efficiency when re-using. |
Besides treating water, photocatalysts have been applied to other fields:
CO Oxidation: Since Carbon monoxide (CO) is extremely harmful to human health, it is important to oxidize it into its much less harmful form, CO2. Photocatalysts can generate radicals / reactive species for this reaction:
CO + 2O2•- + 2H2O → CO2 + 2OH-
Air Purification: If you live near an industrially busy area or place prone to wildfires, air quality is a serious hazard, especially in the long term. Photocatalysts can break down contaminants by installing them in a face mask or air filter by generating the reactive species discussed before.
Hydrogen Gas Production: Hydrogen gas as a clean energy-dense fuel has been at the forefront of news and research recently. Current methods of hydrogen gas, contrary to popular belief, are quite unsustainable. Steam reforming, the most widely-used method for hydrogen production, actually produces H2 from Methane, CH4, which creates carbon dioxide, a greenhouse gas, as a byproduct.
Plastic Breakdown: Photocatalysts are being applied to break down organic pollutants such as plastics. Since plastics are extremely chemically stable, it requires a lot of energy and time for any method to completely break it down. However, photocatalysts have the advantage of being sustainable, non-toxic, and quite affordable.
Conclusion:
Photocatalysts have so many unique advantages that make them a promising candidate for future water purification endeavors, especially in places that need technology that is both effective and cheap. To summarize, when a light source of enough energy hits the photocatalyst, an electron-hole pair is created. This electron-hole pair go on to react with water to form reactive species like radicals. These generated compounds can destroy contaminants and organisms in the water after prolonged exposure.
Author’s Note:
Personally, I am rooting for a breakthrough in photocatalytic water remediation. The research currently being done in photocatalyst optimization is extremely fascinating. For example, scientists have found a way to create single-atom photocatalysts (like independent contractors), which are far more efficient, in a really unique way. At that scale, the properties of the particles are quite different, and it allows the photocatalyst to be much more selective in targeting and breaking down actually harmful contaminants.
Works Cited:
References
Dong, S., Feng, J., Fan, M., Pi, Y., Hu, L., Han, X., Liu, M., Sun, J., & Sun, J. (2015). Recent developments in heterogeneous photocatalytic water treatment using visible light-responsive photocatalysts: a review. RSC Advances, 5(19), 14610–14630. https://doi.org/10.1039/c4ra13734e
Gao, C., Low, J., Long, R., Kong, T., Zhu, J., & Xiong, Y. (2020). Heterogeneous single-atom photocatalysts: Fundamentals and applications. Chemical Reviews, 120(21), 12175–12216. https://doi.org/10.1021/acs.chemrev.9b00840
Loeb, S. K., Alvarez, P. J. J., Brame, J. A., Cates, E. L., Choi, W., Crittenden, J., Dionysiou, D. D., Li, Q., Li-Puma, G., Quan, X., Sedlak, D. L., David Waite, T., Westerhoff, P., & Kim, J.-H. (2019). The technology horizon for photocatalytic water treatment: Sunrise or sunset? Environmental Science & Technology, 53(6), 2937–2947. https://doi.org/10.1021/acs.est.8b05041
Ren, G., Han, H., Wang, Y., Liu, S., Zhao, J., Meng, X., & Li, Z. (2021). Recent advances of photocatalytic application in water treatment: A review. Nanomaterials (Basel, Switzerland), 11(7), 1804. https://doi.org/10.3390/nano11071804
Comments